On April 19th, 1866, Alfred Holt, a Liverpudlian engineer who had apprenticed on the Liverpool & Manchester railroad before taking up steamship design in the 1850s, launched a singular ship that he dubbed the Agamemnon. As the third soon of a prosperous banker, cotton broker, and insurer, he had access to far more personal capital to launch this new enterprise than the typical engineer. This was a lucky thing for him, because the typical investor of the time considered his ambition—to enter the China tea trade on the basis of steam power—foolhardy. A typical oceangoing steamship used five pounds of coal per horsepower per hour and could not compete with sail over such long distances: they would either have to fill most of their potential cargo space with coal or make repeated, costly stops to refuel.[1]

Yet, in the end, Holt pulled off his gamble. He benefited from good timing (perhaps a mix of luck and foresight): the opening of the Suez Canal in 1869 give steamships a tremendous leg up in trade between Europe to the Indian and Pacific Oceans. But in designing ships dainty enough in their coal consumption to pay their way to the Pacific, he also benefited from the late convergence of two complementary developments that had each begun in the early 1800s but did not intersect until the 1850s. First was a series of incremental, empirical improvements to steam engine design: After the massive leap forward from Newcomen to Watt, further increases in steam engine efficiency would be less dramatic. Simultaneously, a theory of heat gradually developed that could explain what made engines more or less efficient, and thus point engineers in the most fruitful direction.
Double-Cylinder Engines
Boulton & Watt erected most of its early pumping engines in Cornwall. Trevithick developed his high-pressure “puffer” there. So, it is only fitting that the last major architectural innovation in piston steam engine design—featuring an entirely new structural component—was Cornish, too. In that region, an ample supply of British engineering talent met an always-eager demand for efficient engines. The ever-deeper mines for extracting metal ore needed ever more pumping power, despite significantly higher coal prices than the coal-rich North.
Joseph Hornblower, born in the 1690s, was one of the first engineers to build Newcomen engines for the mines of Cornwall in the 1720s. Sixty years later, his grandson Jonathan built the first known double-cylinder engine (later called a compound engine). Cornwall’s homegrown natural philosopher, Davies Giddy (later Gilbert), served in the same office he later served for Richard Trevithick, as Hornblower’s scientific advisor. In principle, the idea was quite simple: instead of immediately condensing the remaining steam after the expansion cycle of the piston, the still-warm steam was fed into another cylinder to let it do still more work. However, this added friction, complexity, and cost to the machine. In practice, therefore, Hornblower’s attempted improvement provide no more efficient than a traditional Watt engine.[2]

A generation later, however, another Cornishman took up the idea and carried it further. Arthur Woolf, like many eighteenth-century engineers, got his start as a millwright, but by 1797 was working for the firm of Jabez Carter Hornblower (brother to Jonathan), at a brewery in London, erecting a steam engine. He continued to serve as engineer for the brewery for a decade afterward, and witnessed the operation of Trevithick’s steam carriage in the city in 1803. Woolf realized that he could combine the double-cylinder engine of his former employer’s brother with Trevithick’s truly high-pressure engines (operating at forty pounds per-square-inch or more). The higher-pressure steam, still quite hot after expanding in the first cylinder, would be able to do more work in the second cylinder rather than simply “puffing” out into the atmosphere. Both Watt and Trevithick had (from opposite points-of-view) seen low- and high-pressure steam as rivals, but in Woolf’s machine they complemented one another.[3]
But, as Hornblower had already learned, the path did not always run straight and easy from idea to execution. Woolf led himself astray with an entirely unsound theoretical model for the inner workings of his engine: he believed that steam at twenty pounds per square inch (psi) would expand to twenty times its volume before equaling the pressure of the atmosphere, steam at thirty psi would expand thirty times, and so on ad infinitum. This turned out to be a substantially exaggerated expectation, and led him to begin with a drastically undersized high-pressure cylinder, which let off far too little steam to effectively work its low-pressure mate. Rather than leading him to doubt his theory, the failure of this engine led him into a wild goose chase for a non-existent leak in his pistons.[4]
Woolf’s double-cylinder engine, unlike Hornblower’s, did at last succeed, after years of trial and error, in achieving better efficiency than a Watt engine. But because it was more expensive to build (and thus buy), and more complex to operate, it found favor only in markets without easy access to other, cheaper options. One such example was France, to which Woolf’s erstwhile partner Humphrey Edwards, decamped in 1815: there he sold at least fifteen engines and licensed twenty-five more to a French mining company. Woolf meanwhile returned to Cornwall in 1811, where he found the advantages of his double-cylinder engine soon surpassed by the incremental improvements made by other local engineers to the Boulton and Watt design. He abandoned it after 1824 and built single-cylinder engines until 1833, when he retired to the island of Guernsey.[5]
Meanwhile, steam engine builders carried on with tweaks to get yet one more increment of efficiency out of their engines. They extracted advantages from adjustments to the regulatory machinery of the engine: elements like “release mechanisms,” “dashpots,” and “wrist plates.” The Corliss engine, designed by George Corliss in 1849, became an icon of American industrial design after his company produced a gargantuan specimen to power the 1876 Centennial Exhibition in Philadelphia. Mighty as it was, however, it did not represent a great leap forward in steam engine architecture. Corliss’ design drew its relative advantages over prior engines from a clever combination of previous innovations in the valves that allowed steam to enter and leave the cylinder, and especially in the valve gear that controlled them.[6]
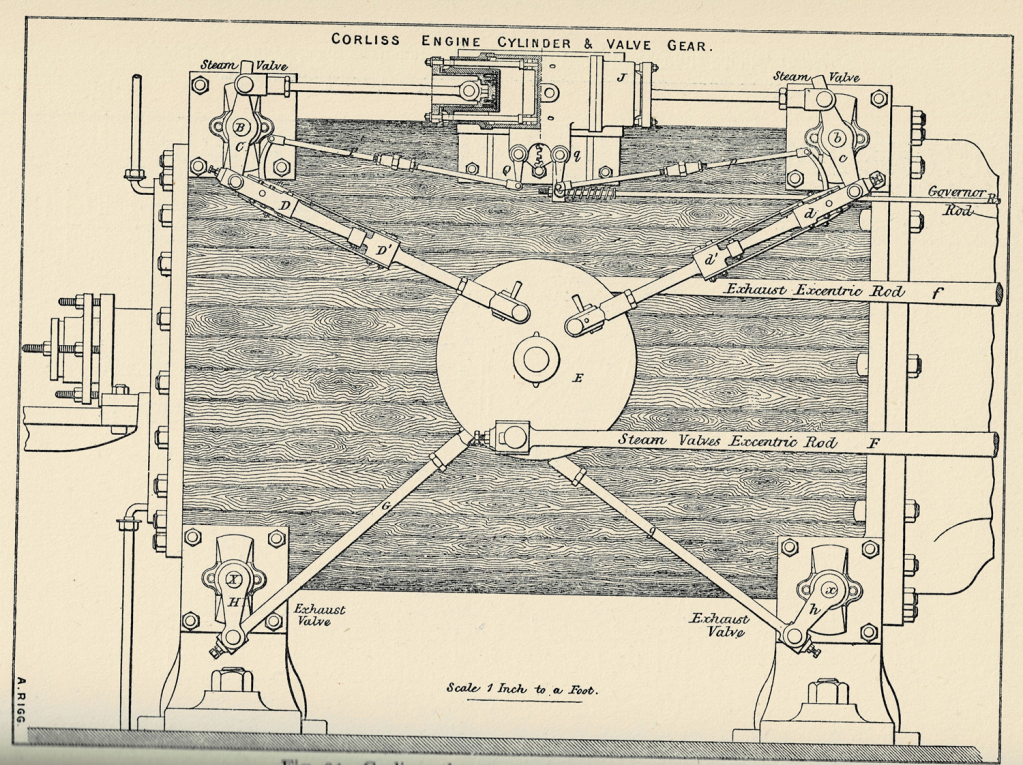
In the meantime, the double-cylinder engine, having failed to prove itself in the 1810s and 1820s, lay dormant. It would be restored to life decades later, by the engineers most desperate to eke as much power as possible out of every ounce of coal: the designers of ocean steamships. But to facilitate the consummation of that match, a solid theory of the steam engine was wanted, one that would dispel, once and for all, the confusions like Woolf’s that continued to trip up engineers’ efforts at improvement.
Measuring Power
The lack of a sound theoretical basis for steam power is evident in the fitful history of cylinder “lagging,” or insulation. Steam engineers borrowed the term lag (a barrel stave) from coopers, because they often insulated early steam boilers with such timbers, held in place with metal straps (this is evident in images of early locomotives like Rocket, with their distinctive wooden cladding).

As early as 1769, Watt had recognized the value of insulating not just the boiler, but also the working cylinder of the engine (emphasis mine):
My method of lessening the consumption of steam, and consequently fuel, in fire-engines, consists of the following principles:—First, That vessel in which the powers of steam are to be employed to work the engine, which is called the cylinder in common fire-engines, and which I call the steam-vessel, must, during the whole time the engine is at work, be kept as hot as the steam that enters it; first by enclosing it in a case of wood, or any other materials that transmit heat slowly; secondly, by surrounding it with steam or other heated bodies; and, thirdly, by suffering neither water nor any other substance colder than the steam to enter or touch it during that time.[7]
Yet, despite Watt’s imprimatur, steam engine builders lagged their cylinders sporadically throughout the first half of the nineteenth century; it was a matter of whim, not principle.[8]
In this era, engineers tended to think of the steam engine as analogous to its predecessor, the water wheel. Steam replaced liquid water as the mechanical working fluid, but just as water drove the wheel by pushing on its vanes, in their minds steam performed work by expanding and pushing on the piston. A typical description of the time stated that “[t]he force of the steam-engine is derived from the property of water to expand itself, in an amazing degree, when heated above the temperature at which it becomes steam.”[9] Engineers knew that the cylinder ought to be kept hot to prevent condensation of the steam inside, but within this framework it was not obvious that it ought to be kept as hot as possible. Watt, emphasizing the contrast between the hot cylinder and the cool condenser, had drawn attention to the role of heat in the engine, but the introduction and success of high-pressure engines with no condenser, where the primary factor seemed to be the expansive force of steam, muddled matters once again.
The gradual development of a new, more robust theory began with a practical problem: how to measure the amount of power an engine generates. This became a particularly pressing problem for Boulton & Watt in the late eighteenth century, as they expanded from the traditional business of pumping engines into the new market of driving cotton mills. The traditional way of measuring the output of a steam engine, in terms of “duty” (the pounds of water lifted by one foot per bushel of coal burned) had gradually been supplemented with the concept of “power,” typically expressed in horsepower: pounds lifted over a given distance, but over a given period of time rather than with a given amount of fuel. Thomas Savery had begun to grope towards the concept in his 1702 book on the virtues of his steam pump, The Miner’s Friend:
I have only this to urge, that water, in its fall from any determinate height, has simply a force answerable and equal to the force that raises it. So that an engine which will raise as much water as two horses working together at one time in such a work can do, and for which there must be constantly kept ten or twelve horses for doing the same, then, I say, such an engine will do the work or labour of ten or twelve horses…[10]
Note here that Savery proposes to measure the muscular equivalent of the engine not in terms of the output of just the pair of horses running the machinery, but in terms of the total stock of horses that a mine owner would require to maintain the same power over a long period of time. This model of horsepower in terms of economic equivalency did not stick, however, and by the late eighteenth century horsepower became fixed to Watt’s figure of 33,000 foot-pounds per minute.
Yet this remained a measure of power best suited to pumping work: if a mine needed to raise 20,000 pounds of water per hour from a 200-foot-deep shaft, one could readily calculate the engine horsepower required. Cotton spinning machinery—which varied in size, function, and design—did not lend itself to such simple arithmetic. In order to properly size engines to mills, Boulton & Watt needed some way measure the horsepower produced by an engine while driving various combinations of machinery.
From the beginning, Watt had attached gauges to his engines to measure the pressure inside the engine, by connecting a small indicator cylinder to the main engine cylinder so that steam could flow between them. The level of pressure in the indicator could serve as a proxy for power output. But to actually capture the data was a maddening exercise, because the pressure varied constantly as the piston worked up and down. A means of capturing this continuous data came from a long-time Watt employee, John Southern. He had joined the company as a draftsman in 1782, and despite a predilection for music that the strait-laced Watt found suspicious, quickly became indispensable.[11]

In 1796, Southern devised a simple device to solve the power measurement problem. He attached a piece of paper above the indicator, rigged so that it would move back and forth as the main piston operated. Then he attached a pencil to the tip of the pressure gauge. As the pressure went up and down, so would the pencil, while the paper moved left and right beneath it with the cycle of the engine. The result, when running smoothly, would be a closed shape, which Southern called an indicator diagram, and the averagepressure during the operation of the engine could be computed from the average distance between the top and bottom lines of that shape, which would in turn be proportional to the power. By calibrating the diagramwhile an engine was pumping water, where the power output was well-defined, Boulton & Watt could then determine the power produced by the same engine while operating a given set of mill machinery.[12]

Thermodynamics
Engineers now had a tool at hand for diagnosing the internals of a running engine. That tool, in turn, provided the seed for the birth of the science of thermodynamics, which began as the science of the steam engine. The first great leap in that direction was made by Sadi Carnot.
Carnot’s story carries more than a whiff of the tragic. Though later honored as a founding father of thermodynamics, he achieved no recognition in his lifetime, and died of cholera as a still-young man in 1832. His father Lazare was an accomplished engineer and a major political figure in revolutionary France, but what we know of the son comes almost entirely from a fifteen-page biography sketched decades after the fact by his younger brother Hippolyte, which begins, pathetically, with the statement that: “the life of Sadi Carnot was not marked by any notable event…”[13]

In fact, Carnot’s short life was remarkably eventful. He grew up in Napoleon’s court, attended the elite engineering school École polytechnique at age 16, and was at the Chateau Vincennes during the 1814 assault on Paris that ended Napoleon’s first reign. He returned to Paris as a staff lieutenant in 1819, filling his free time with his passions: music, art, and scientific studies. There, in 1824, he produced his seminal work, Réflexions sur la puissance motrice du feu (Reflections on the Motive Power of Fire). In it he endeavored to explain how heat produces motion. I will allow him to elaborate in his own words:
Every one knows that heat can produce motion. That it possesses vast motive-power no one can doubt, in these days when the steam-engine is everywhere so well known. To heat also are due the vast movements which take place on the earth. It causes the agitations of the atmosphere, the ascension of clouds, the fall of rain and of meteors, the currents of water which channel the surface of the globe, and of which man has thus far employed but a small portion.[14]
As we have seen, the tendency of engineers to conceive of steam hydraulically, as a fluid that generated work through pressure much like water in a water wheel, had engendered some confusion about how to build and operate an engine most efficiently. Ironically, Carnot moved the understanding of the steam engine forward by taking the analogy of a steam engine to a water wheel even more seriously than his contemporaries. However, for him the key power-generating agent was not the pressure of steam, but the fall of heat. Just as a waterwheel required a head from which water descended by gravity to turn the wheel, so the steam engine required a reservoir of high heat, which then flowed down to a cold body and thereby did work.
For Carnot this fall of heat in a steam engine was quite literal: it consisted of an imponderable fluid called caloric, that drained out from the hot body to the cool one:
The production of motion in steam-engines is always accompanied by a circumstance on which we should fix our attention. This circumstance is the re-establishing of equilibrium in the caloric; that is, its passage from a body in which the temperature is more or less elevated, to another in which it is lower. …The steam is here only a means of transporting the caloric.[15]
This caloric theory of heat as a substance still predominated in Carnot’s day, despite subversives like Count Rumford who advocated for a mechanical theory of heat, which understood heat purely as a form of motion.
If the flow of heat from the hot to the cold body produced all the work in the steam engine, then making an efficient engine meant minimizing any spillage of heat that did no useful work. It also implied that to maximize the work produced by the engine, one must maximize the difference between the source of high temperature and the sink of low temperature—the height through which the caloric fluid falls.
Carnot’s book was largely ignored. But his insights had their first chance to be rescued from obscurity shortly after his death. Émile Clapeyron, just a few years younger than Carnot, was an accomplished engineer who specialized in locomotives, and a fellow-graduate of the École Polytechnique. In 1834, he published a paper in the school’s journal showing that Carnot’s heat engine theory could be expressed in the language of calculus and seen graphically in the indicator diagram: the area inside the diagram (which could be expressed as an integral) corresponded to the work performed by the heat transfer in the engine. Clapeyron’s work revived Carnot’s abstractions, put them on a firmer mathematical basis, and publicized them to the community of engine builders. Yet once again, they reached a dead end. Steeped in the traditions of their craft, neither Clapeyron nor his peers seem not to have understood the heat engine theory as having practical applications to real-life engineering.[16]
Vindication for Carnot would have to wait another fifteen years, when a series of exchanges between William Thomson (later Lord Kelvin), Rudolf Clausius, and James Joule shortly before and after 1850 resolved various problems with the Carnot-Clapeyron heat engine, including reconciling it with the mechanical theory of heat: what flowed from the hot to the cold body was not a literal fluid but an abstraction called energy, which could take on many forms, but could only perform useful work over a fall in temperature. Through the medium of energy, a certain quantity of heat was directly equivalent to a certain amount of power.[17] The scientist who best synthesized this new science of heat for a wider engineering audience was Thomson’s colleague at the University of Glasgow, Macquorn Rankine.
Perfecting the Marine Engine
Rankine’s position was something of a novelty: he was only the second person to hold a chair of Civil Engineering at Glasgow, a position established by Queen Victoria in 1840. From the days of Watt and beyond, the University of Glasgow had been more practical-minded than the great Oxbridge schools of the South. But the establishment of a faculty chair in engineering did not just indicate that the university supported more hardheaded tasks than absorbing classical learning, it also signaled a desire to elevate engineering into a more theoretical, scientific discipline.[18]
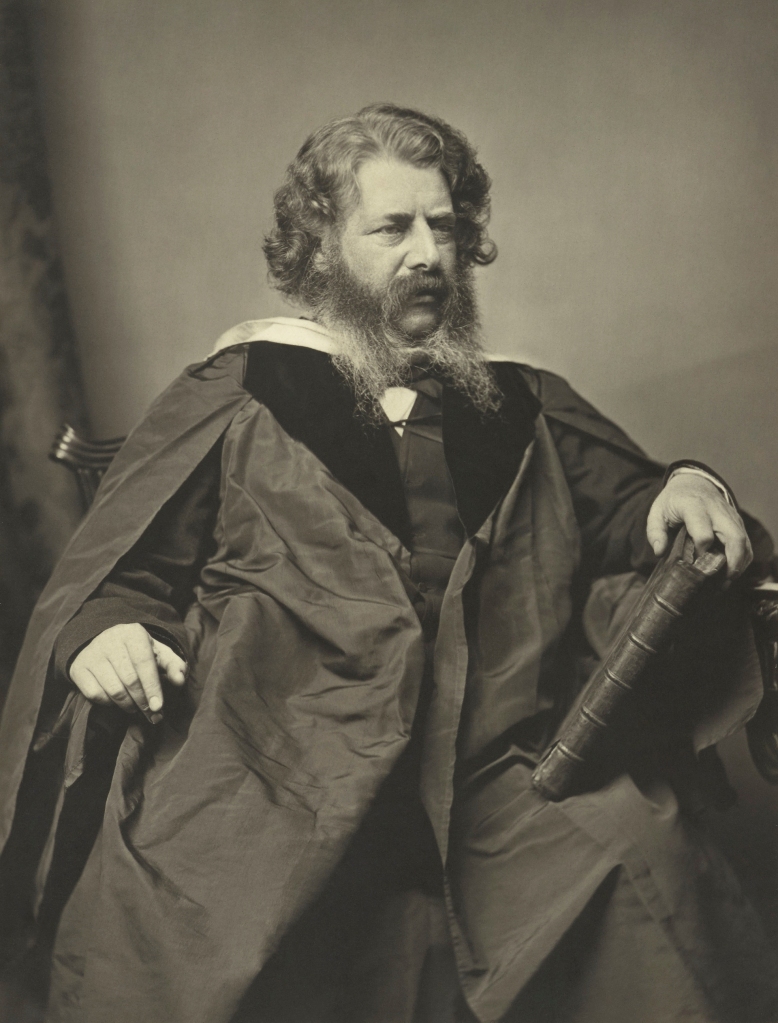
Rankine, embodying this new spirit, straddling the worlds of theory and practice, preached thermodynamics to the engineering world: his 1859 A Manual of the Steam Engine and Other Prime Movers (1859), a 500-page, densely mathematical treatise, explicated the new theory and its applicability to practical matters in great detail and popularized the term “thermodynamics.” However he also knew how to reach a wider audience: in an 1854 address to the Liverpool meeting of the British Association for the Advancement of Science (BAAS) he concisely expressed the laws of thermodynamics in terms of ordinary English and simple arithmetic: “As the absolute temperature of receiving heat is to the absolute temperature of discharging heat, so is the whole heat received to the necessary loss of heat.” That is, the more precipitous the fall of temperature from the high (receiving) to the low (discharging) point of the engine cycle, the more efficient the engine could be.[19]
Among those in Rankine’s circle of influence in the 1850s was an experienced builder of marine steam engines in Glasgow named John Elder, who became the first to incorporate a double-cylinder engine into a successful steamship. Elder had marine engines in his blood: his father David had joined Robert Napier’s engine building firm and began designing steamboat engines in 1821. In addition to family tradition and his natural talents, Elder had two other advantages in this undertaking. First, he had access to Glasgow’s “thermodynamic network” (as the historian Crosbie Smith put it); he had tutors in the new thermodynamic science and probably got specific advice from Rankine to introduce steam jacketing to prevent condensation in the cylinder. Second, he had an eager buyer.[20]

The Pacific Steam Navigation Company (PSNC) of Liverpool had overextended itself in the South American Pacific-coast trade, where high-quality steam coal could arrive only by a 19,000-mile round-trip supplied by sail. Profit margins were slim to none, and venture stayed in the black only by virtue of a government mail contract. This made the company willing to wait out teething problems in order to get a more efficient engine. From the time Elder and his partner took out their engine patent in January 1853, it took four years before PSNC ratified the superiority of their ship Valparaiso, which consumed 25% less coal than an equivalent single-cylinder model.[21]
Elder’s success set the stage for Holt’s further vault forward in the 1860s. Among the latter’s achievements was to convince the Board of Trade that marine engines could operate safely at higher pressures; allowing a greater fall of temperature and thus more efficient use of fuel. This, in turn, set the stage for triple-expansion engines later in the century, to extract still more work from the heat as it falls from boiler to condenser. This polyphonic fugue of machinery heralded the age of steam’s baroque period, which engendered the fantasias of steampunk a century later. By about 1890, a triple-expansion engine, running at 160 pounds-per-square-inch, could consume one-and-a-half pounds of coal per-horsepower per-hour, less than a third of the going rate a few decades before, and about five times less than Watt’s engine.[22]
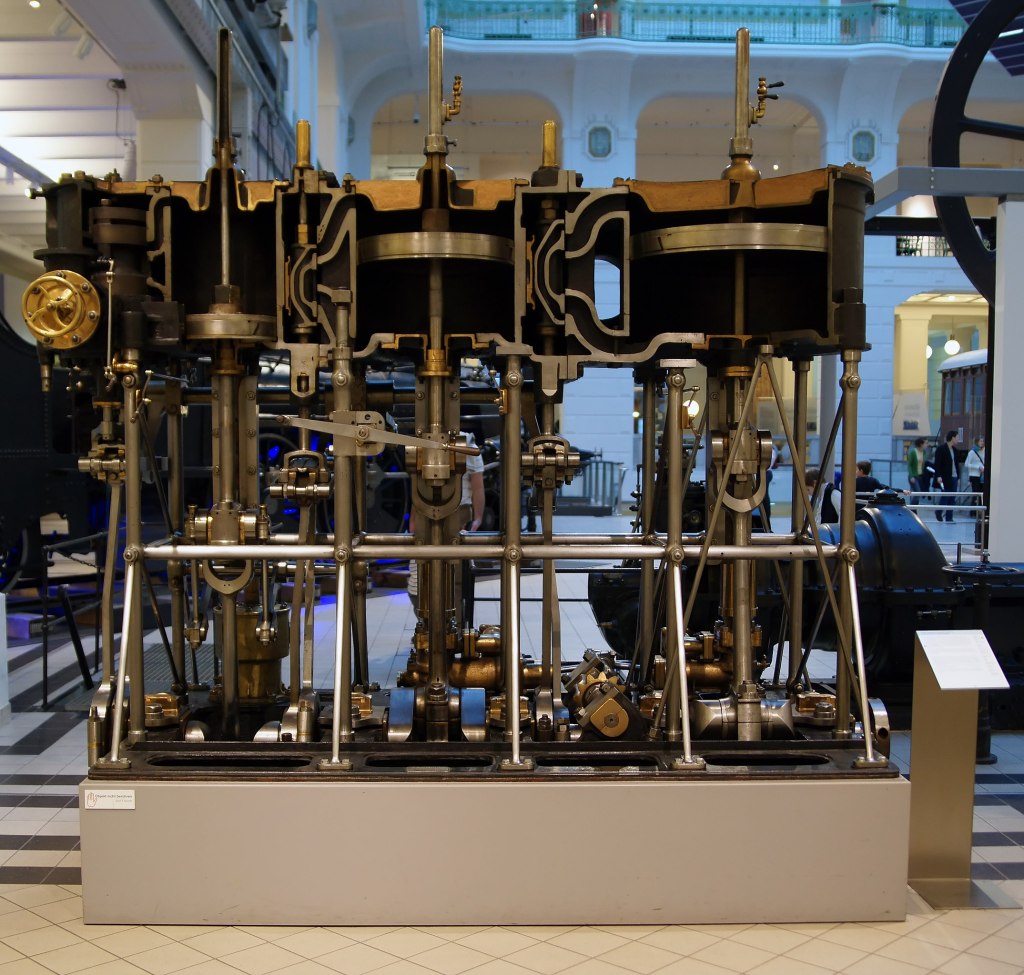
Yet even as it thrust the age of steam up towards its apex, thermodynamics pointed out the weak spot that would lead to its downfall. In his 1854 speech to the BAAS, Rankine had touted the advantages of the air engine, a device devised by the Scotsman Robert Stirling that used hot air as its working fluid. As Rankine pointed out, the laws of thermodynamics have nothing in particular to do with steam, but hold “true for all substances whatsoever in all conditions…” Air had a decided advantage over steam insofar as it could be driven to very high temperatures without creating very dangerous pressures: “For example, at the temperature of 650 ° Fahr. (measured from the ordinary zero,) a temperature up to which air engines have actually been worked with ease and safety, the pressure of steam is 2100 pounds upon the square inch; a pressure which plainly renders it impracticable to work steam engines with safety….”[23] The Stirling air engine did not, in the event, prove to be the slayer of steam. Its use never expanded beyond occasional low-power domestic applications. But it brought the first adumbration of the coming eclipse.

[1] Francis E. Hyde, Blue Funnel: A History of Alfred Holt and Company of Liverpool from 1865 to 1914 (Liverpool: Liverpool University Press, 1956), 1-19.
[2] John Farey, A Treatise on the Steam Engine (London: Longman, Rees, Orme, Brown, and Green, 1827), 384-392; Hills, Power from Steam, 147-148; D.S.L. Cardwell From Watt to Clausius: The Rise of Thermodynamics in the Early Industrial Age (Ithaca: Cornell University Press, 1971), 78-79.
[3] Rhys Jekins, “A Cornish Engineer: Arthur Woolf, 1766-1837,” Transactions of the Newcomen Society 13, 1 (1932), 55-56.
[4] Rhys Jekins, “A Cornish Engineer,” 57-58.
[5] Rhys Jekins, “A Cornish Engineer,” 59-63.
[6] Hills, Power from Steam, 178-184.
[7] Quoted in James Alfred Ewing, “Steam Engine,” Encyclopedia Britannica, vol.25 (Cambridge: Cambridge University Press, 1911), 820.
[8] Hills, Power from Steam, 236-267.
[9] Quoted in D.S.L. Cardwell, “Science and Steam in the Early Nineteenth Century Reconsidered,” Transactions of the Newcomen Society 49, 1 (1977), 111.
[10] Thomas Savery, The Miner’s Friend (London: W. Clowes, 1827 [1702]), 26.
[11] H.W. Dickinson, James Watt: Craftsman and Engineer (Cambridge: Cambridge University Press, 2010 [1935]), 131.
[12] Hills, Power From Steam, 91-93.
[13] Sadi Carnot (R.H. Thurston, ed.), Reflections on the Motive Power of Heat (New York: John Wiley & Sons, 1897), 20.
[14] Carnot, Reflections on the Motive Power of Heat, 37-38.
[15] Carnot, Reflections on the Motive Power of Heat, 44-45.
[16] Milton Kerker, “Sadi Carnot and the Steam Engine Engineers,” Isis 51, 3 (September 1960), 260-263.
[17] Hunt, Pursuing Power and Light, 37-40.
[18] “Department of Civil Engineering,” Records of the University of Glasgow, Scotland (https://archiveshub.jisc.ac.uk/search/archives/5423a1c4-bcfe-3c21-8dcd-0ebf353a9207?component=0b5e6a0f-6850-3a0f-8aa9-27b1d6c59d23); R. A. Buchanan, “The Rise of Scientific Engineering in Britain,” The British Journal for the History of Science 18, 2 (July 1985), 218-233.
[19] W.J. Macquorn Rankine, “On the Means of Realizing the Advantages of the Air-Engine,” Journal of the Franklin Institute, 60, 4 (October 1855), 253.
[20] Crosbie Smith, “Witnessing Power: john Elder and the Making of the Marine Compound Engine, 1850-1858,” Technology and Culture 55, 1 (January 2014), 76-106; David F. Channell, “The Harmony of Theory and Practice: The Engineering Science of W. J. M. Rankine, ” Technology and Culture 23, 1 (January 1982), 48
[21] Smith, “Witnessing Power,” 86-87, 89, 102.
[22] Adrian Jarvis, “Alfred Holt and the Compound Engine,” in Robert Gardiner, ed., The Advent of Steam: The Merchant Steamship Before 1900 (London: Conway Maritime Press, 1993), 156-159; Hills, Power from Steam, 241-242.
[23] Rankine, “On the Means of Realizing the Advantages of the Air-Engine,”
Here Here! More interstellar work. Try sending to Smithsonian magazine for publication.
LikeLike